Lighting the Spark
Harvard signals its investment in quantum science
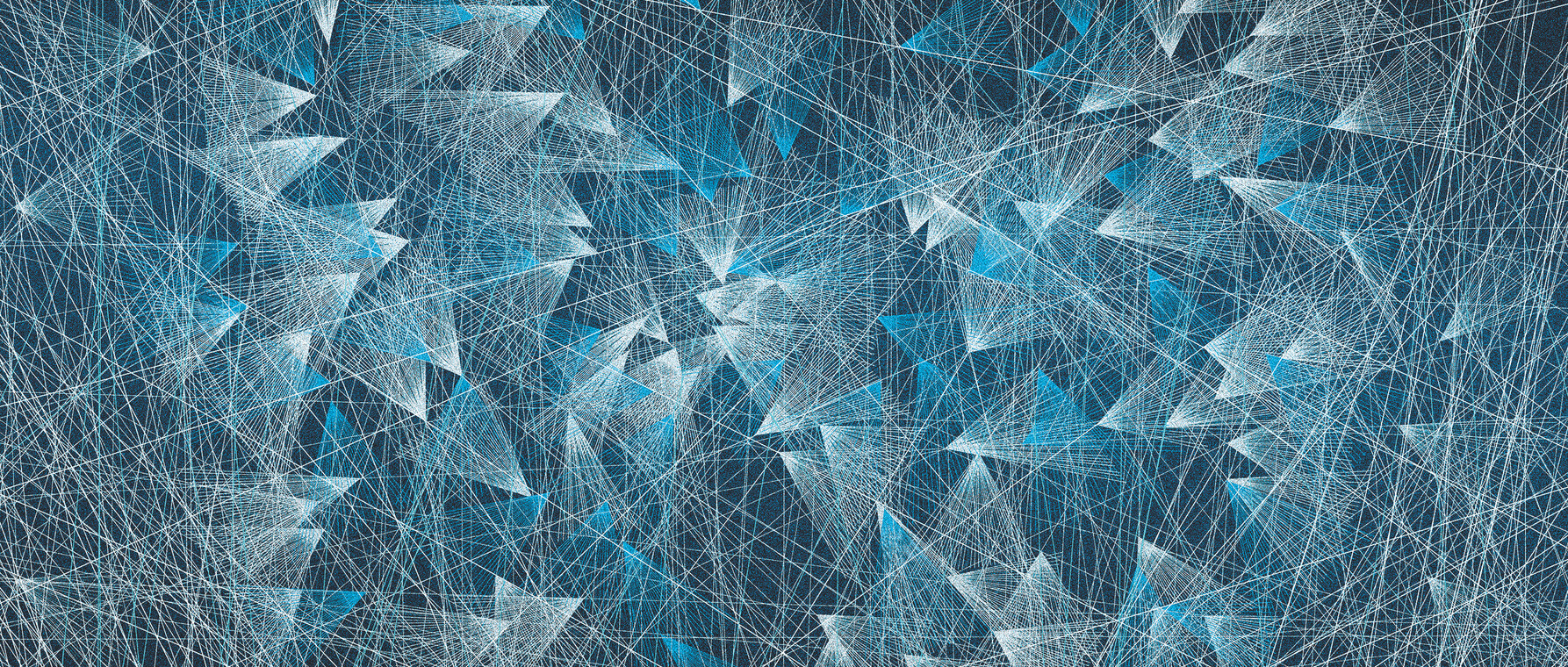
Imagine, for a moment, that you could forget everything you ever knew about the way our reality works. That two objects hundreds of miles apart could communicate instantaneously. That the act of measuring something could change what is being measured. That something—a cat, perhaps—could be both alive and dead at the same time.
But this actually is the way our reality works. Or at least it does at the microscopic level. When you look at smaller and smaller objects— objects at the atomic and subatomic levels—quantum effects seem to make interactions between objects operate differently than predicted by the kind of classical physics most of us learned in high school. The quantum world is so counterintuitive, so strange, that even Max Planck (who coined the term quanta) searched for an alternative explanation for his results.
More than a century later, quantum theory may seem mysterious to the lay person, but mathematically, it’s 100 percent calculable. “Quantum mechanics tells you what is going to happen, very precisely,” says John Doyle, Henry B. Silsbee Professor of Physics in the Faculty of Arts and Sciences (FAS). “When you do the calculations, there’s no mystery about it at all. The mystery is only that microscopic physics behaves differently than what we see in our daily lives.”
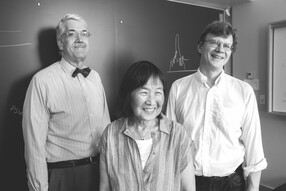
Doyle is one of the co-directors of the Harvard Quantum Initiative (HQI), a cross-disciplinary community dedicated to expanding research, development, and education on quantum information and its possibilities. Launched in November 2018, HQI forms part of a larger effort in the United States to advance understanding of quantum science and technology and initiate the development of innovative materials and devices for high-speed computing, networking, and ultra-precise sensing. Doyle and his colleagues—co-directors Evelyn Hu, Tarr-Coyne Professor of Applied Physics and of Electrical Engineering at the Harvard John A. Paulson School of Engineering and Applied Sciences (SEAS), and Mikhail Lukin, George Vasmer Leverett Professor of Physics at FAS—see the advancement of quantum science as a radical effort to expand scientific boundaries and rethink how research is accomplished.
“We believe that quantum science represents a profound change in the understanding of what information is, which will affect everything we do moving forward,” says Hu. “That change will affect how we measure, how we compute, and how we communicate.” By embracing the physics that operates at the quantum level, researchers can tackle problems—or reinvestigate old ones—in groundbreaking new ways that hold transformative potential. “For people in the sciences, this is a tremendous opportunity to create novel applications and instrumentation that can be used to further enable scientific research,” Hu says.
We believe that quantum science represents a profound change in the understanding of what information is, which will affect everything we do moving forward.
–Evelyn Hu
Hu deliberately uses the term “quantum science” and “quantum engineering” instead of “quantum physics.” While early breakthroughs in quantum science came from theoretical physicists, the next generation of quantum scientists has come from physics, computer science, chemistry, and mathematics. This broader collaboration is expected to, in turn, accelerate and enrich progress in these fields, but in ways that cannot at the moment be easily predicted. “Breakthroughs often happen at the interfaces of traditional disciplines,” says Lukin.
Spooky Action up Close
Those breakthroughs will rely on a strong foundation of basic science, built in part by faculty, graduate students, and recent graduates from Harvard. Srujan Meesala, PhD ’19, is emblematic of the young scientists whose work sits at the intersection of multiple fields. Meesala studied electrical engineering as an undergraduate, and though he wanted to focus on physics for his graduate work, he’s grateful for the hands-on experience he brings to his research. “There is a need for engineers who think about making devices to study interesting physics,” he says, “and for people from hard physics backgrounds to become more engineering oriented.” Meesala brought his expertise to the lab of Marko Loncar, the Tiantsai Lin Professor of Electrical Engineering at SEAS.
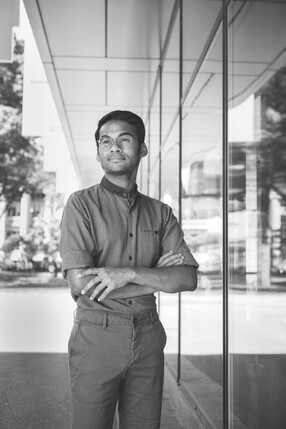
Meesala wanted to discover how to use quantum effects to transfer information. To conduct this research, he’s using sound and flaws in an unlikely source— diamonds. “My research uses defects inside diamonds, called color centers,” he explains. Diamond crystals are made of carbon atoms. By removing one carbon atom and replacing a second with another element—nitrogen or silicon, for example— he can create an individual quantum system inside a solid. “This is interesting for quantum information applications because you can store and process information at the level of the atom inside a solid.”
Experiments on color centers have shown certain quantum effects, such as when two quantum particles interact and become interdependent, a process known as entanglement. Einstein found the phenomenon so startling that he famously called it “spooky action at a distance.”
“Entanglement is hard to observe in nature. You need to engineer very specific quantum systems to see it,” Meesala explains. “Isolating and manipulating these individual defects is one way to do that.” By taking advantage of color centers, researchers have measured the entanglement of particles in two diamonds located a kilometer apart by using the light emitted by the defects.
But while quantum entanglement has been generated between diamond color centers at a distance, it is hard to achieve between two defects in the same diamond. “To perform local quantum operations with these color centers, we would ideally put a number of color centers on one chip and have them interact.”
Entanglement is hard to observe in nature. You need to engineer very specific quantum systems to see it."
–Srujan Meesala, PhD '19
Meesala used sound in an effort to induce these interactions. “You can quantize sound at the quantum level into particles called phonons,” he says. “I wanted to determine if we could get one color center to interact with another by exchanging sound waves.” In their experiment, Meesala and his colleagues carved a thin string out of diamond, then applied voltage to stretch it and study how sound waves couple to a defect called the silicon vacancy center. They envisioned that sound can move along such a string like a pressure wave, causing some of the atoms to compress and others to elongate, essentially transferring information from defect to defect.
Meesala was able to conclude that a silicon vacancy center provides a good system for testing the sound-mediated transfer of quantum information, which could be applied in quantum computers and networks. The work was published last year in Nature Communications.
Along the Way
Di Wei, PhD ’19, has taken a different approach to solving the problem of information transfer at the quantum level, but one still grounded in basic science research. As an undergraduate working in a condensed matter lab at the University of Denver, she connected with scientists like her whose inquisitiveness drove them to solve problems for their own sake. “I’m of the school of thought that you should do basic research for the sake of basic research, and that technology will arise from that,” she says. “Special relativity is my favorite example of that because it’s so abstract, but it is the reason we have technologies like GPS today.”
These energizing collaborations fueled her decision to pursue graduate studies at SEAS, where she continues to satisfy her curiosity about physics. Wei joined the lab of Amir Yacoby, professor of physics and of applied physics at FAS and SEAS, where she found numerous opportunities to engage in fundamental research questions. One of Yacoby’s projects involved looking for spin superfluidity in graphene, a two-dimensional sheet of carbon atoms.
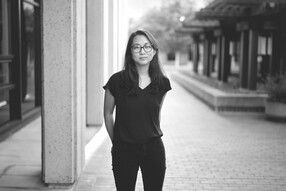
“Spin superfluidity is a magnetic analog to superconductivity, using the electron’s spin as carriers of information as opposed to their charge,” Wei explains. “You can think of each spin as a local magnetic moment that can interact with neighboring spins. If the spin at one site is tilted, its reorientation is felt by nearby spins.” This tilting creates a spin wave that can transfer angular momentum from site to site, which can be used to send information with less heat than is currently generated by traditional electrical methods used in computerized devices. “A superfluid of spins would allow for the transfer of spins with no energy loss, which would be a fundamentally new kind of system to study,” she says.
Yacoby and colleague Bertrand Halperin, Hollis Professor of Mathematics and Natural Philosophy, Emeritus, had postulated that superfluidity could be found in graphene, and Yacoby and Wei set out to test this theory. Using a type of magnetic material known as a quantum hall ferromagnet, they observed some intriguing physics that could help measure exotic quantum states via a process called electron interferometry. “The first thing we saw was an interesting interference pattern between electrons,” Wei says. “The pattern confirmed that graphene was a good platform for electron interferometry.” This work was published in Science Advances in August 2017.
Next, Wei used an electric current to generate spin waves through the graphene and was able to detect those waves via an induced voltage. “It’s the first transport measurement of spin waves in a graphene quantum hall ferromagnet,” Wei says. “They were predicted to exist in this system but were difficult to detect with electronic methods because they are excitations of an insulating material.” In October 2018, she was lead author on a paper about these findings, which appeared in Science magazine.
Although Wei wasn’t able to demonstrate spin superfluidity with certainty, her results do establish graphene as worthy of further study. “Often, the original idea that researchers set out to test doesn’t work, but they are still able to find interesting things along the way,” she says.
A Changing World
Doyle, Hu, and Lukin believe that Harvard is well positioned to make advancements in quantum science and engineering through the work of researchers like Meesala and Wei—large enough to tap existing intellectual prowess and small enough to act on ideas and move more quickly that other institutions. “A new discipline is being created right in front of our eyes,” says Doyle.
“It’s obvious that this is a special time,” Lukin continues. “We have a very special community of world-leading researchers. Harvard is home to the most powerful quantum machines ever made.” He notes that hundreds of young leaders who trained at Harvard are now conducting their own cutting-edge research at institutions around the world, pushing the boundaries of what is currently known about the quantum realm.
And while this new discipline holds tremendous potential—so much so that Harvard, the US, and the world are willing to invest in its promise—it’s impossible to anticipate what the ultimate impact will be. “No inventor could predict how the world would use their invention,” says Hu. “We don’t know where the spark will be lit, a spark that will burn a pathway to new applications.”
Art by Kai and Sunny, photos by Kris Snibbe, John Soares, and Thomas Broening
Get the Latest Updates
Join Our Newsletter
Subscribe to Colloquy Podcast
Simplecast