At the Quantum Frontier
Improving accuracy to make the next generation of computing a reality
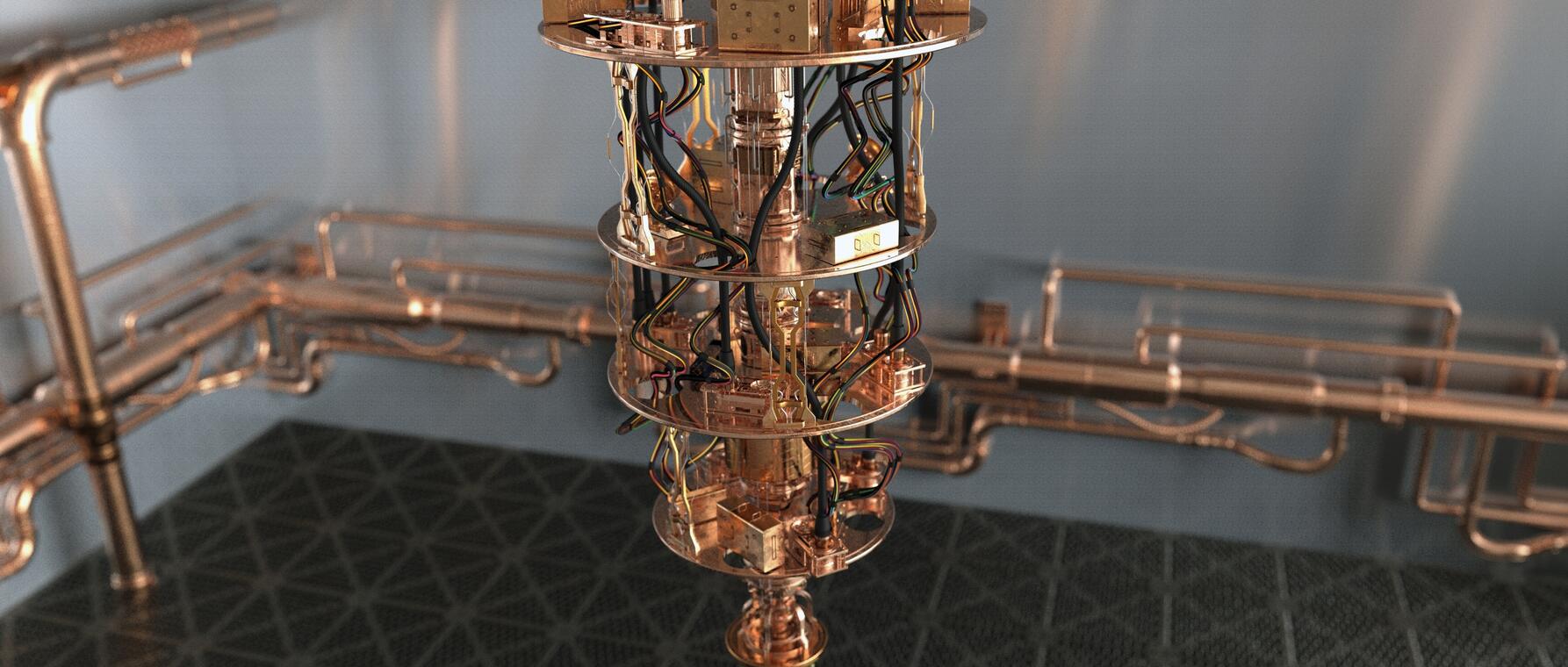
Quantum computers have the potential to revolutionize our understanding of the world around us—and teach us how to manipulate it. The technology could enable the rapid design and development of life-saving drugs, simulate superconducting materials that would revolutionize technology and clean energy, and even offer insight into the underlying structure of space and time. Like the qubits that sit in superposition at the heart of quantum computers, the possibilities seem endless.
“Right now, you will find people who see quantum computing as a panacea,” says Susanne Yelin, a professor of physics in residence at Harvard’s Faculty of Arts and Sciences. “I am not one of them. But quantum computing could help us better understand fundamental physics, such as problems in condensed matter or particle physics. It could also advance quantum chemistry [which uses quantum physics to understand chemical systems]—and with it, better development of drugs and materials.”
At the Harvard Kenneth C. Griffin Graduate School of Arts and Sciences (Harvard Griffin GSAS), PhD physics students Maddie Cain, on whose dissertation committee Yelin sits, and Dolev Bluvstein are working to make the promise of quantum computing a reality. In the laboratory of Professor Mikhail Lukin, Cain and Bluvstein push the boundaries of science, advancing the prospects of transformative applications that could reshape our world.
Power and Potential
Quantum mechanics refers to the physics of things at a very small scale—like atoms and electrons. One of its characteristics is superposition, where things can exist simultaneously in two states. This property is one of the foundations of quantum computing. Classical computers like the laptop on which you may be reading this story represent information as zeroes and ones (basically, current switching off and on in a circuit). Every “bit” of information can only be a zero or a one. A quantum computer, however, uses qubits that can exist in superposition as zero, one, or any linear combination of the two.
Quantum computing could . . . advance quantum chemistry [which uses quantum physics to understand chemical systems]—and with it, better development of drugs and materials.
—Professor Susanne Yelin
Bluvstein, Cain’s colleague in the Lukin lab, provides a tangible example of superposition: "Let’s say there’s a bottle of water on a table,” he says. “In the classical universe, that bottle stays on the table, but a quantum bottle of water could exist in two places at the same time. This sounds like nonsense, but when you zoom in on things and see them get smaller and much colder, the physics that we use to describe the world changes completely."
Another characteristic of quantum computers is entanglement. When qubits are entangled, any change in the state of one produces a corresponding change in the state of the other—both across time and space.
The potential of a computer that leverages the laws of quantum physics lies in its ability to process information at speeds unimaginable with classical computers. "By exploiting superposition and entanglement, quantum computers can solve certain problems exponentially faster than regular computers, which makes them extremely powerful,” says Bluvstein. “They give us a completely new computational tool for understanding the universe, which is in fact quantum itself."
To Err Is . . . Quantum
This immense computing power comes with its own set of challenges, particularly in maintaining the delicate states of qubits, which are highly susceptible to unwanted environmental interactions and, therefore, error.
That’s where Cain’s research comes in. Her work focuses on two critical areas: understanding the power of quantum computers relative to classical computers and developing efficient methods for error correction.
"The properties of superposition and entanglement make the quantum bits extremely fragile, and interactions from their environment cause errors that are costly to fix," she says. "In addition to better understanding the relative usefulness of quantum and classical computers, I’m trying to find out better ways to correct errors."
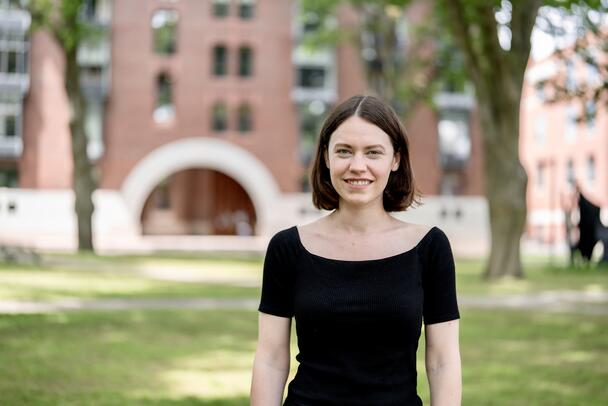
Cain’s work matters because the great challenge of quantum computing is converting all that superpositional possibility into real, usable information. Qubits collapse into a state of zero or one like classical bits as soon as they’re measured. To make sure the measurement yields useful information, quantum computers use quantum gates—akin to the logic gates used to facilitate operations in classical machines—to create “interference” and manipulate the qubits to achieve the desired measurement result. But quantum systems are fragile, so if the logic gates have errors, the measurement will be off. So how can quantum engineers make sure that their machines yield a correct answer to a question?
Maddie’s recent work on correlated decoding and algorithmic fault tolerance provides fundamental new insights into fault-tolerant quantum computation and results in practical improvements of error-corrected algorithms by several orders of magnitude.
—Professor Mikhail Lukin
The answer lies in the use of logical qubits, made by combining many individual qubits to make the information more reliable and to help detect and correct errors that might happen during computations. “It's like having redundancy to ensure accuracy and fix mistakes if they occur,” Cain explains.
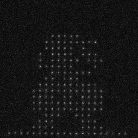
The possibility of quantum error correction is one of the main reasons to take seriously the promises of quantum computation. Many researchers have proposed viable, clever, and effective ways to deliver nearly unique signatures of the quantum errors but translating from the signatures to error-free results—a process called “decoding”—is itself a difficult problem.
Lukin, the Joshua and Beth Friedman University Professor and co-director of the Quantum Science and Engineering Initiative, says Cain’s theoretical work has opened up completely new research directions in quantum algorithm development and quantum error correction.
“Maddie’s recent work on correlated decoding and algorithmic fault tolerance provides fundamental new insights into fault-tolerant quantum computation and results in practical improvements of error-corrected algorithms by several orders of magnitude,” he says. “In the long term, this work will likely have a transformative impact in the field of quantum information science and technology.”
A Dynamic New Approach
To have quantum computing, of course, you have to have qubits. Bluvstein works to create them through the innovative use of neutral trapped atoms. "In my research, I use single rubidium atoms that are trapped by laser beams,” he says. “These beams act as tweezers to snatch the atom. Once the atom is trapped, we can make them very cold so that they start to behave in a quantum way. We can then use the atoms as qubits.”
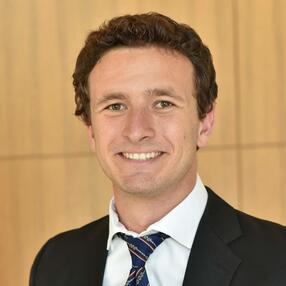
Bluvstein’s method produces qubits that can maintain their quantum states for extended periods—crucial for performing reliable quantum computations. "The electrons of the quantum atoms, once they have been zapped with the laser beams, start getting excited and interact with the electrons of other quantum atoms,” he says. “By carefully arranging these interactions, we can program quantum computations and use the atoms as a quantum computer."
Bluvstein and his collaborators also came up with a different method for designing quantum computers in which the connections between parts of the device can be changed while it's running. (Imagine if you could rearrange the roads in a city at rush hour while cars were driving on them to make traffic flow better.) Lukin, his Bluvstein’s faculty advisor, says Bluvstein’s work could be a game changer for quantum computing.
By carefully arranging the interactions [between electrons of quantum atoms], we can program quantum computations and use the atoms as a quantum computer.
—Dolev Bluvstein
“Dolev and his collaborators developed a completely new approach involving the so-called reconfigurable quantum computer architecture,” Lukin says. “It enables us to change the connectivity of a quantum processor dynamically during the computation process. Most recently, this approach enabled major advances in the realization of quantum error correction and the so-called fault-tolerant quantum computers—quantum computers that are robust to errors. This work completely transformed the frontier of the field and has emerged as the most promising approach to building large-scale quantum computers.”
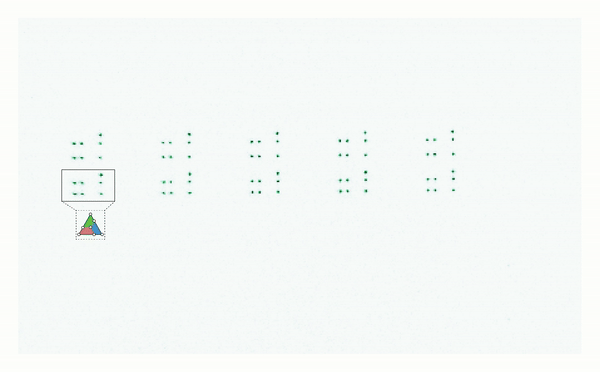
Promise and Challenges
Quantum computing heralds a future full of promise but also challenges. One of the big concerns for Cain is the technology’s potential impact on data encryption. "Today, most of our data is encrypted, like my credit card information,” she says. “Encryption relies on the fact that it is really hard for a classical computer to factor numbers. But a quantum computer could break in days the kind of encryption it might take a conventional supercomputer many years to decipher. So, there are a lot of people thinking about how we can make our information safe once quantum computers become accessible."
At the same time, Cain says that quantum computers could revolutionize fields like materials science and energy. "Quantum computers allow us to simulate quantum mechanics efficiently since they rely on those principles,” she says. “This allows us to study materials that can help us in our everyday lives, such as superconductors. If we could figure out a way to conduct electricity at higher temperatures efficiently, we could save a lot of energy. A powerful way to discover those materials is through simulating them. A quantum computer helps make those simulations easier to run."
Bluvstein, too, is optimistic about the future and the ongoing quest to harness the power of quantum mechanics: "Right now, I’m looking forward to going back to the lab. I’ll see my friends and we’ll do some experiments on our atoms together.”
Both Cain and Bluvstein credit the collaborative environment at Harvard Griffin GSAS as a significant factor in their research success. "What makes Harvard Griffin GSAS so special is that it brings together some of the best young scientists around the world,” Bluvstein says. “It’s these collaborations that have allowed us to make amazing advances in quantum mechanics."
This supportive, generative environment has enabled Cain to push the boundaries of quantum computing. "I have been able to be a part of several major experimental advances, even as a theorist," she says. "Just knowing that I am working on one of the leading platforms for quantum computation is really exciting because we know the questions that we need to answer, we know what is next. We are pushing the field forward."
This research was funded by the National Science Foundation, the U.S. Department of Energy, and the U.S. Army Combat Capabilities Development Command Army Research Laboratory.
Get the Latest Updates
Join Our Newsletter
Subscribe to Colloquy Podcast
Simplecast