Q+A: Colleen Cavanaugh
A path-breaking biologist whose imaginative research has transformed how we understand the microbial foundations of symbioses and whose discoveries have contributed new insights into evolution at the organism to genome level.
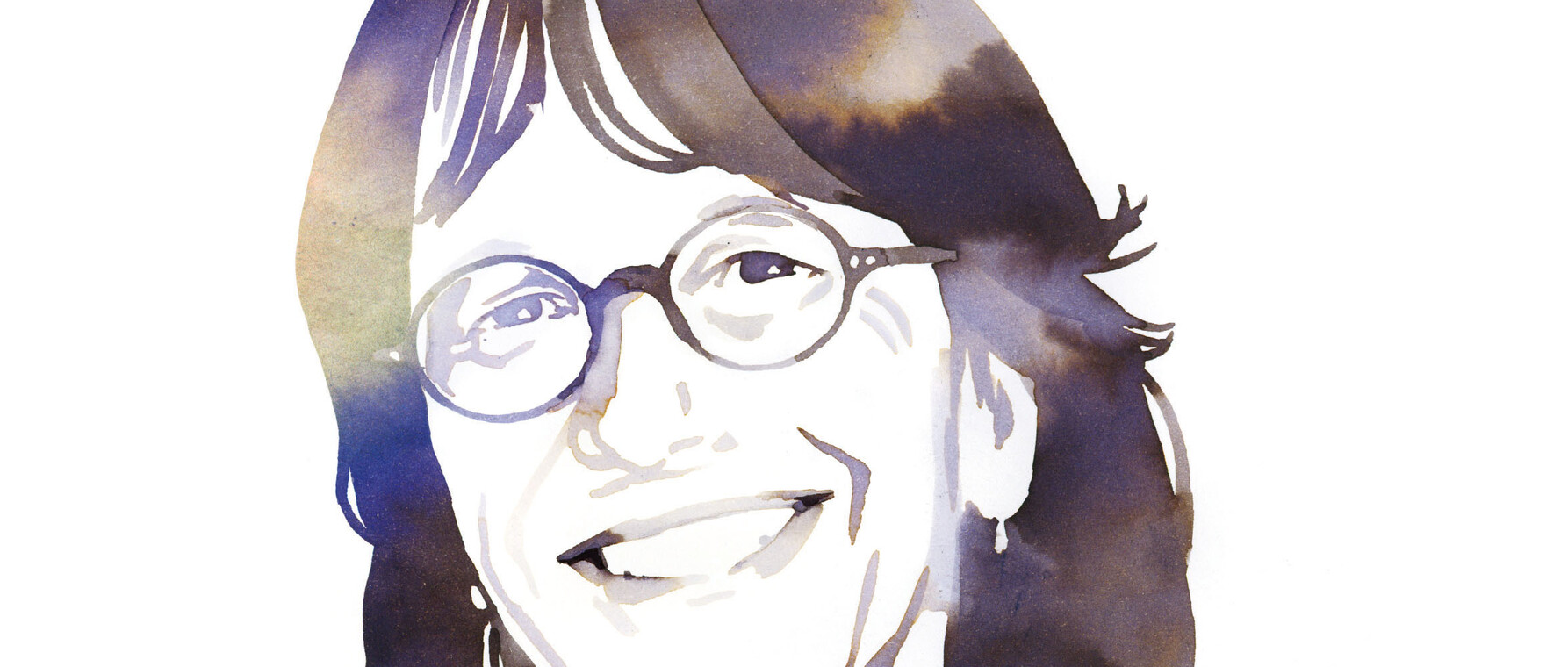
NAME: Colleen Cavanaugh, PhD ’85
FIELD OF STUDY: Biology
TODAY: Edward C. Jeffrey Professor of Biology and Co-Director of the Microbial Sciences Initiative
Colleen M. Cavanaugh’s ground-breaking work explaining the biochemistry of symbiosis between giant tube worms living amidst hydrothermal vents deep on the ocean floor and the one-celled bacteria that live inside them transformed how scientists understood the symbiotic role that bacteria play in chemosynthesis. Her research illuminates the microbial dimensions of symbioses and also has important evolutionary implications. Cavanaugh’s work demonstrates that, like chloroplasts in plant cells, which evolved from free-living bacteria, chemosynthetic bacteria produce food for their animal hosts by using sulfur compounds instead of sunlight as an energy source. Her current research focuses on symbiont transmission strategies—how symbiotic partnerships are maintained generation to generation, and the effects on genome evolution, which provide new clues for understanding how hosts and symbionts co-evolve.
Cavanaugh received her BGS in biology from the University of Michigan before earning a PhD in organismic and evolutionary biology from Harvard in 1985. Cavanaugh is Edward C. Jeffrey Professor of Biology in the Department of Organismic and Evolutionary Biology and co-director of the Microbial Sciences Initiative at Harvard. She is a fellow of the American Association for the Advancement of Science and the American Academy of Microbiology and an adjunct Senior Scientist at the Bay Paul Center of the Marine Biological Laboratory in Woods Hole, Massachusetts.
What drew you to science?
I knew from a young age I was interested in science. For many people who go into biology research one of the main entryways is fieldwork—and that’s how it was for me. As an undergraduate at the University of Michigan, I took a course on marine ecology, followed by a six-week, U of M-sponsored course in Woods Hole, Massachusetts, that also tapped into scientists at the Marine Biological Laboratory (MBL) and the Woods Hole Oceanographic Institution (WHOI). We had two weeks of lecture, followed by four weeks to design and execute a research project, write it up, and present it. At Michigan I had written a paper on Limulus—horseshoe crabs. I had learned that they mate on the full moon—what biologists call lunar periodicity, so I decided to test this out. It didn’t go exactly like the existing scientific literature said it would. I searched beach after beach on the full moon, never finding them, until I finally met a fisherman/invertebrate zoologist, who told me “when the lilacs bloom, the Limulus spawn.” It turned out to have as much to do with temperature as with tides. Eventually, I did find them; I did counts throughout many a cold May night. Coming up with the project—figuring out how to collect the data, getting the data, then analyzing it—that was incredible. That’s when I saw firsthand that there were things that were not known in science. And that’s when I fell in love with research and with Woods Hole.
What brought you to Harvard?
A pure love of bacteria. I wanted to learn more about what bacteria were doing in the “real” world—outside the lab, that is. At the MBL I worked with John Hobbie, an aquatic ecologist, who was devising novel ways of studying bacteria in natural samples and this opened up new ways of studying them. At Michigan, I had focused on ecology and that became important to my studies of bacteria as well. Everything is a habitat for bacteria, whether it’s water, soil, sediment, or other organisms, including humans. At Harvard, I had advisors in both fields. James J. McCarthy, who is now my colleague, and who co-chaired the Nobel Peace Prize–winning Intergovernmental Panel on Climate Change, is a biological oceanographer, and Ken Sebens, now at the University of Washington, is a community ecologist.
Intellectually, there’s a bit of serendipity in how you acquired the various different research experiences that turned out to play a formative role in your thinking about chemosynthesis and symbiosis.
At the time, my decision to study any particular topic or question never felt like a conscious decision. Many of my first research experiences came from working on various projects in Woods Hole prior to graduate school. My initial introduction to microbial processes was working as a research assistant at the MBL’s Ecosystem Center, helping with experiments on an oil spill in the Cape Cod Canal. Then I worked with Hobbie, who made important advances in how we can see, and therefore, count bacteria using fluorescence microscopy. It turns out we underestimated—by orders of magnitude—how many bacteria were in water, in sediment, and in soil. And this sense of scale opened up a new window onto the entire field of microbial ecology. I was also interested in nutrient cycling, the constant, fundamental cycling of elements in biological systems, in my case, ocean and coastal systems.
Then, in my first year of graduate school I took a course called “Nature and Regulation of Marine Ecosystems,” taught by my advisors and Ruth Turner, a pioneering deep-sea marine biologist. We learned to read scientific papers critically; the deep-sea hydrothermal vents had been recently discovered, and there were a series of talks on them. Turner started off with the ecology of animal communities, and Holger Jannasch, from WHOI, talked about chemosynthetic bacteria as the base of the food chain. Finally, Meredith Jones, curator of worms at the Smithsonian, described the giant, red-plumed tubeworm. The question was how were they eating, since they are mouthless and gutless. He mentioned the presence of sulfur crystals in their tissue. This was a clue for me—when I was at Woods Hole they were working on the sulfur cycle, and sulfur is an energy source for chemosynthesis. So I raised my hand and said, “It’s perfectly clear—they must have chemosynthetic sulfur-oxidizing bacteria as symbionts inside their tissues.” Then I convinced him to send me some tissue samples. And I started to try to prove that there were bacteria in them.
This hypothesis, that bacteria living inside giant tubeworms were the symbiotic mechanism for chemosynthesis, was at first met with skepticism. You had to work very hard to do the science to prove it. How did that experience shape they way you approached your subsequent research?
When I started this research I had yet to take a microbiology course, so I had to learn on my feet and figure out what I needed to learn. Three things stayed with me. The first I learned from Jannasch, who was the expert on vent microbiology. He was skeptical and put me through my paces—but gave me just enough encouragement to keep me going. His skepticism taught me to be critical and rigorous in my analysis and to always include controls in my experiments, both positive and negative. The other legacy of that experience reflects my natural temperament: I was determined, thorough, and wanted to learn from the foundation up. I went and talked to everybody I could—I was and am a sponge when it comes to knowledge. In my own research, with my students, and postdocs, I’m a bit of a “control” freak. In microbial ecology, we work with organisms that are abundant in the environment, yet invisible to the naked eye, so it’s especially crucial to be extremely rigorous in experimental design. Moreover, it’s essential to have a thorough understanding of your field, investigate that subject to the utmost, and simultaneously, be in the lab trying things.
How did your own experience as a graduate student at Harvard shape how you teach your own students?
I want my students to understand that not everything is known. In all of the courses I’ve taught, and that includes my freshman seminar on the biology of symbiosis, I always have a research paper component. It’s important that students learn there are many questions left to ask. When students read a scientific paper, I want them to think like they are a part of the lab group that wrote it and then I ask them, “What would you do next, and why is that important”? “What’s next?” is always the question.
What’s next?
I’m interested in co-evolution, the relationship of bacteria and animals, and how symbiosis evolves over time: initially, you have two or more completely different organisms, somehow they get into proximity with each other, there may be an initial conflict, but somehow they reach détente, and form stable relationships—that benefit at least one of the partners. Recently, we have been studying transmission strategies: once established, it’s important in evolutionary terms to maintain a stable symbiosis over generations. But how does that work? In symbiosis, bacteria are transmitted vertically, from mother to offspring, or horizontally, when the host acquires the symbionts from the environment or a contemporary. By sequencing entire genomes of symbiont populations, we have identified mixed methods of transmission, which have implications for genome evolution. In translational terms, these findings will help us analyze and track the biogeographical distributions of pathogens, whether they are traveling as free-living microbes or conveyed by a host. This research continues to expand our understanding of the full range of symbioses. More broadly, everything in the field of microbial symbioses, suggests that such relationships, ranging from mutualistic to parasitic associations, are far more prevalent than currently recognized.
Get the Latest Updates
Join Our Newsletter
Subscribe to Colloquy Podcast
Simplecast