Freedom of Movement
Hamilos considers the brain, the origins of action, and the question of free will.
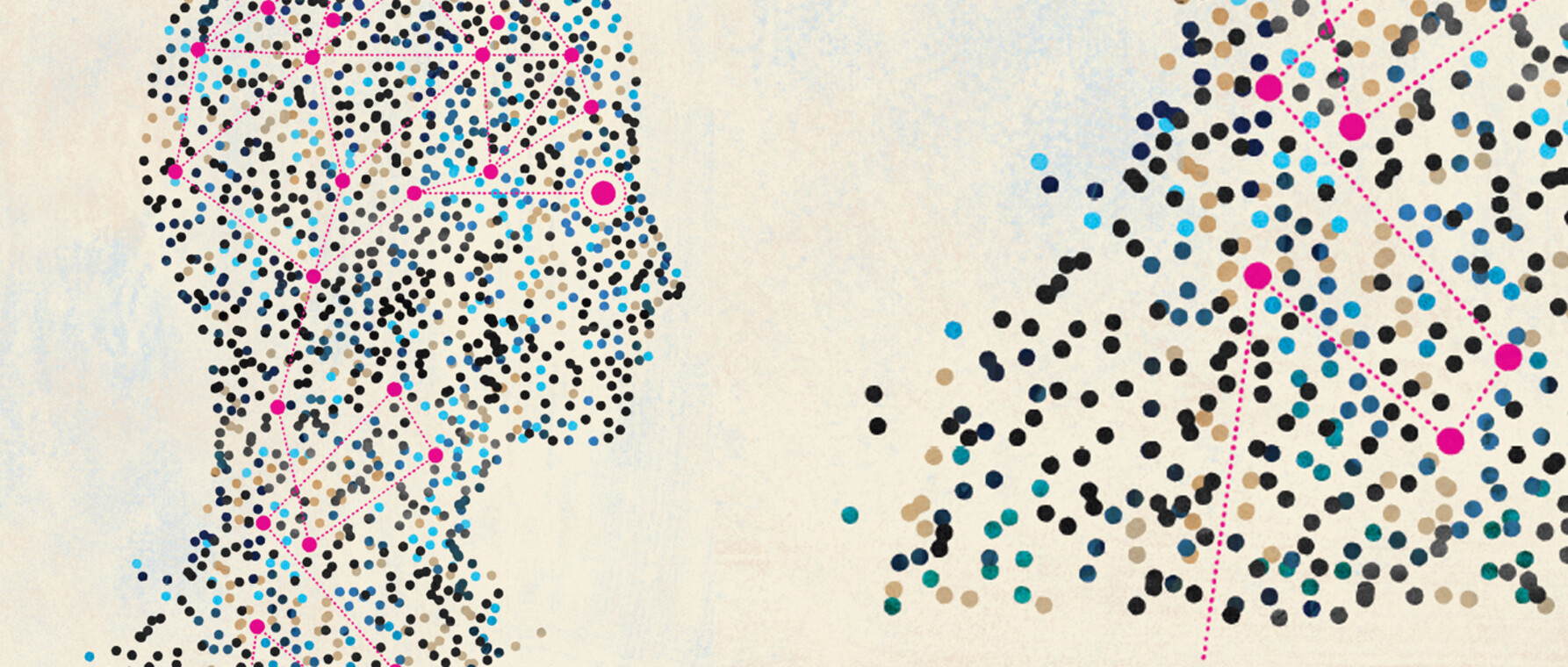
Research at Risk: Since World War II, universities have worked with the federal government to create an innovation ecosystem that has yielded life-changing progress. Now much of that work may be halted as funding is withdrawn. Find out more about the threats to medical, engineering, and scientific research, as well as how Harvard is fighting to preserve this work—and the University's core values.
It’s morning. Maybe you’re reading this article at your kitchen table. You’ve got a cup of coffee there. You take a sip almost without thinking as you read. Why? Yes, it’s your favorite blend and God knows you need the caffeine. But why did you bring the cup to your lips at that exact moment? Why not a second after—or a second before?
“We know we can react to sensory triggers like dodging speeding cars in the crosswalks of Harvard Square,” says neuroscientist Allison Hamilos. “But introspection tells us that many—if not most—of what we do arises from our own volition; that we can decide to do things like pick up our coffee without obvious external prompting. What goes off in that black box between our ears that lets this happen? Where does spontaneous action come from?” The question of what “makes us move” has fascinated and stumped philosophers for centuries. Today, Hamilos— who received her PhD in medical sciences from Harvard’s Graduate School of Arts and Sciences in 2021—studies the neurological processes behind voluntary motion. Working with Professor of Neurobiology John Assad, PhD ’91, the recent alumna sheds new light on the role played by the neurotransmitter dopamine in self-initiated action. In so doing, she is also challenging the basis for how we understand ourselves and our relationships with others: the idea that human beings have free will.
Searching for the Soul of Action

Attempts to explain the origin of action from physical phenomena go back at least to the 17th-century French philosopher and scientist René Descartes, who described the body as a machine that could react to its environment in ways not so different from our modern understanding of the way our reflexes work. But Descartes had no physical explanation for the origin of voluntary behavior and so reasoned it must arise from some non-corporeal “soul.” Even with the advent of modern neurobiology, Hamilos says we continue to grapple with this mystery.
“The 20th-century physiologist Sir Charles Sherrington pioneered our understanding of neural reflex arcs—the pathways in the brain that control our reflexes,” she says. “Stimuli like contact with a hot stovetop trigger our bodies to move—unconsciously—to more advantageous positions. But even though Sherrington believed voluntary actions arose from a physical source, he didn’t have an explanation for how this works, either, and believed finding their ‘spring of action’ would be essential to truly understanding what makes us who we are.”
Now, in the 21st century, armed with unprecedented genetic and computational tools to observe and manipulate the brain, Hamilos is part of a new generation of neuroscientists poised to discover the physical origins of volition. To begin her exploration, she and her team of researchers draw on insights from Parkinson’s disease. Sufferers notoriously have trouble with tremors and movement—but not all movement. It may be a challenge for a seated Parkinson’s patient to stand up or to reach for a glass and drink, for instance, but throw an object at them and they can dodge it with surprising agility. Because Parkinson’s results from the degeneration of dopamine neurons, the paradox of motion in patients suggests the neural “circuits” involved in self-generated movement are different than those that enable us to dodge impatient drivers or catch footballs.
“We think the issues with movement in Parkinson’s have to do with the loss of dopamine neurons from a tiny strip of the brainstem called the substantial nigra pars compacta,” Hamilos explains. “When you look at the brain of a Parkinson’s patient, you can see these cells are pretty much gone. That’s why we suspect these neurons might be part of Sherrington’s ‘spring of action.’”
Dopamine, a neurotransmitter that facilitates communication between brain cells, likely matters for spontaneous movement because of its interaction with an evolutionarily ancient brain circuit called the basal ganglia. Hamilos suspects the basal ganglia acts like a kind of amplifier for the brain’s higher-reasoning center, the cortex. When cells of the motor regions of the cortex fire in the right patterns, it causes muscles to contract and enables movement. What causes these cells to fire? Dopamine plays a key role.
Our brains are already setting us up to do something, that suggests we don’t choose what we do. Ultimately, our spontaneity might all come down to some kind of randomness that’s going on in the brain—in other words, things we can’t control.
—Alison Hamilos, PhD ’21
“There are positive and negative ‘feedback circuits’ in the basal ganglia that send signals to the motor regions of the cortex,” Hamilos explains. “Dopamine stimulates the positive feedback circuits and suppresses the negative ones. For this reason, dopamine is poised to increase activity in the cortex’s motor regions, and this could help the brain decide both if and when to move. So, our hypothesis was that less dopamine would mean less positive drive to the cortex, less cortical firing, and less spontaneous action. It’s how we think we get the movement phenomenon of Parkinson’s. If your cortex isn’t able to ‘rev up’ its own activity as well as normal, it might be more difficult for you to initiate new behaviors like picking up a cup.”
Of Mice and Movement
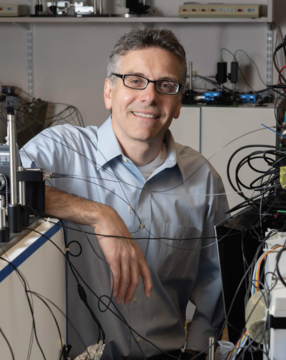
Given that the loss of dopamine neurons disrupts voluntary action in those who have Parkinson’s disease, does the normal signaling of these neurons facilitate spontaneous movement in those who are healthy? To test this hypothesis, Hamilos performed an experiment on mice trained to make spontaneous movements in a self-timing task. First, Hamilos gave the mice a sip of Gatorade from a spout positioned in front of them exactly 5 seconds after a cue in the form of a flash of light and a sound. Repeating the process many times, she conditioned the mice to anticipate the treat after the visual and aural cues; the mice started licking the spout even before the juice came.
“This is how we coax the animals into timing their movements,” she says. “The mice anticipate the Gatorade and start to sip before the juice comes—just like Pavlov’s dog.”
Next, the mice received Gatorade for making a voluntary movement: a lick at least 3.3 seconds after a cue.
“We rewarded the mice for spontaneously initiating their licks when we wanted them to,” Hamilos says. “If they licked between 3.3 to 5 seconds after the cue, we gave them Gatorade immediately. But if they licked too early or too late, they didn’t get any treats on that trial and had to wait several seconds before getting to try again. ”
Although the animals adapted to the new circumstances and started to selftime all their licks relative to the cue, the timing of their movements was highly variable over many trials. Sometimes the mice moved too early. Other times they moved too late.
“In theory, the thirsty mouse should be motivated to time their licks accurately,” she says. “But they don’t. That suggests they can’t perfectly time their movements. We suspected that the firing of dopamine neurons might explain why a mouse moved a little earlier or later on a given trial in this timing task.”
To find out how the signaling of dopamine neurons related to the movement timing of the mice, Hamilos introduced an otherwise harmless virus into the animals that made their brain cells glow green when dopaminergic firing increased. Then, she looked at the glowing signals in the brains of the mice through tiny fiber-optic “telescopes” to see what the dopamine neurons were doing while the animals timed their movements. She found that the dopamine neurons sent timer-like signals to the basal ganglia in the interval between the cue and the movement. Right after the cue, the signals started at a low level and built up slowly, peaking just before the mice moved.
“It’s as though reaching that peak level communicated to the brain precisely when to move,” Hamilos says.
More surprising, perhaps, was the finding that the neural signals could actually predict when the mouse would move even before the cue happened “When dopamine neurons were more active before the cue, their signaling ramped up faster, and the animal moved relatively early on that trial,” Hamilos says. “But when dopamine neurons were less active before the cue, their timer signal rose more slowly, and the animal only moved once it had peaked—later than usual.”
To see if dopamine neurons could control when movement initiated, Hamilos and her team did another experiment using optogenetics—a technique that involves shining light on genetically modified neurons to control the strength of their signaling in awake, behaving animals. When the researchers increased the signaling of dopamine neurons during the self-timing interval, the mice consistently moved earlier. When they suppressed the signaling, the mice moved later.
“Together, this shows that the signaling of dopamine neurons explains both when and why mice decide to move when they do,” Hamilos says. “It suggests that these neurons might be something like the physical embodiment of Descartes ephemeral soul in the brain—at least as far as deciding when to do something is concerned.”
Randomness or Free Will?
HMS Professor John Assad, Hamilos’s dissertation advisor, helped her to develop the idea for her project and to review and analyze data. The two, along with collaborators Giulia Spedicato, Hong Ye, Fangmiao Sun, and Yulong Li, published the findings from the experiments in two papers currently undergoing peer review. Assad says that movement initiation is still a mystery, but Hamilos’s research brings neuroscientists one step closer to understanding the mechanisms behind it.
“Some hints, like Parkinson’s disease, for instance, have suggested that dopamine neurons in the brain may be involved in self-initiated movements, but their role has remained unknown,” Assad says. “Allison’s clever self-timed movement behavioral task in mice showed that the activity of dopamine neurons predicted when movement would occur even seconds in advance. These results point to the dynamics of dopamine neurons as a critical signal for self-initiated movements, and further provide a view of how their loss in Parkinson’s patients could lead to difficulty in initiating movements.”
But if dopamine neurons help initiate activity, what initiates them? Why do we do what we do?
Hamilos says scientists don’t know the answer to that question, but she suspects the brain activity that caused the pre-cue dopamine signals in her mice could have several different ultimate causes—signals from other brain areas representing thirst, boredom, or fatigue, for example. It’s also possible that this activity maybe completely random. Heat changes the excitability of neurons, for instance, so it could be that moment-to-moment temperature fluctuations in dopamine neurons predisposed her mice to be in different states of readiness for action before the cue. It could be that recent experiences and learning predisposed the mouse to time its movement differently, trial to trial. Whatever the case, Hamilos acknowledges that her research is “a bit unsettling” because it challenges some of our notions of free will.
“When I was young, I always thought of free will as meaning we make our own choices—no higher power or deterministic universal construction forces us to do anything,” she says. “But as I’ve gotten older, I’ve started to ask what it would mean practically to say we get to choose? On the one hand, if the dopaminergic signals we observed before the timing cue mean our brains are already setting us up to do something, that suggests we don’t choose what we do. Ultimately, our spontaneity might all come down to some kind of randomness that’s going on in the brain—in other words, things we can’t control.”
At the same time, Hamilos says that free will could mean acting on the basis of the feelings, desires, dreams, and experiences that make us who we are. Those qualities may be encoded in our brain cells, neurotransmitters, and neural pathways, but there is still a “self”—even if it’s not in our conscious control—and it does make decisions.
“So, if we think of this ‘self ’ as who we are,” she says, “people with a set of personality traits, wants, needs, likes, and dislikes that arise in the circuits of the brain as the result of our genetics and our experiences, and if this ‘self ’ predisposes your brain to behave in a certain way... if you think about it that way, then in that sense, I think we do have free will.”
This research was funded by the National Institutes of Health.
Illustration by Keith Negley; Photos by Tony Rinaldo and Anna Olivella
Get the Latest Updates
Join Our Newsletter
Subscribe to Colloquy Podcast
Simplecast