Colloquy Podcast: A Faster, Greener Way to Meet the World’s Limitless Demand for Data
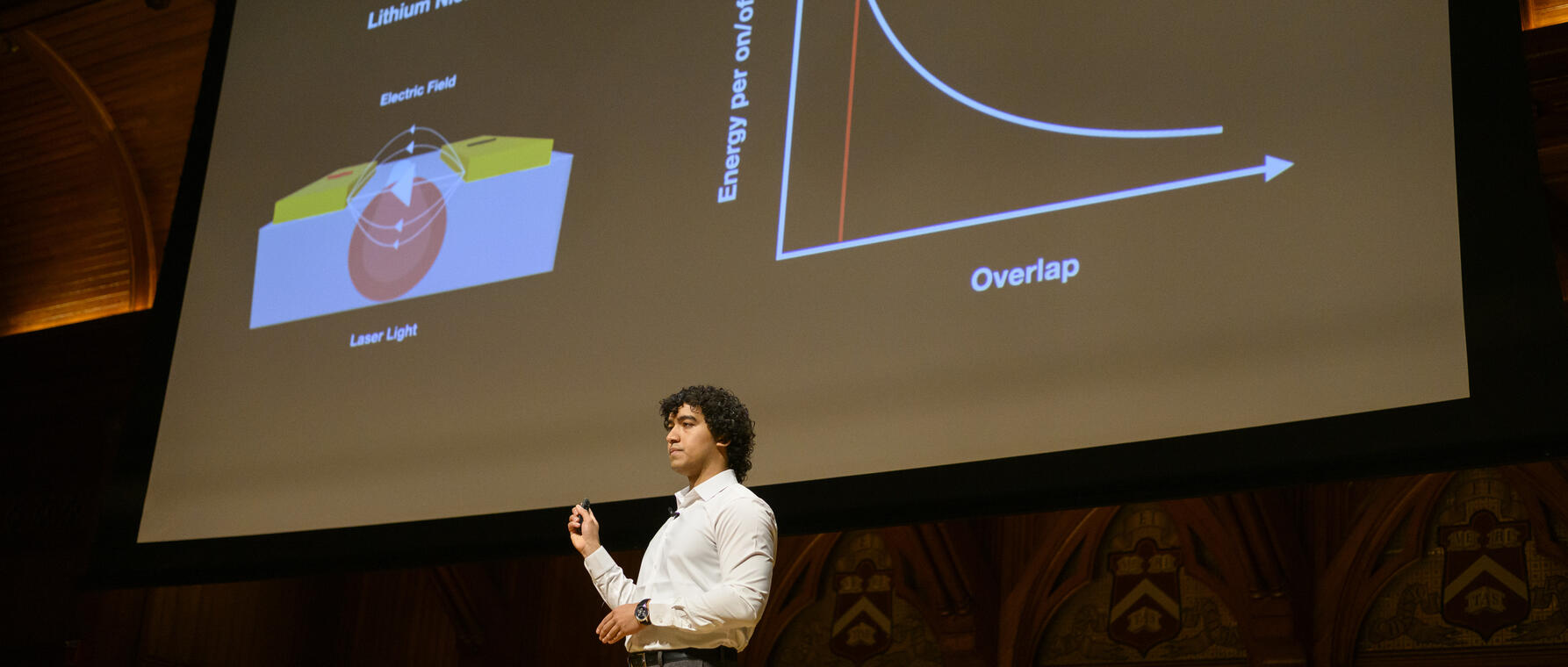
Humanity generated over one septillion bits of data this past year alone. All that information takes energy to transmit. Lots of energy. In fact, data-associated technology could account for up to 20 percent of global energy production by 2030. In this talk given at the 2024 Harvard Horizons Symposium, physicist Dylan Renaud, PhD ’24, describes how using light at the nanoscale level may be a way to meet the almost limitless need for information while meeting the planet’s need for sustainable practices.
This transcript has been edited for clarity and correctness.
We live in a world interlaced with our data. From the cameras filming me now to the text I sent my brothers just a few moments ago, data is all around us and we just can't seem to get enough of it.
If we look at just how much data is created across the globe each year, we see something shocking. Over just the past few years, over 90 percent of humankind's digital data has been created, with over 1 septillion bits this past year alone. That's one followed by 24 zeros. For scale, that's about as many stars as there are in the observable universe. And this growth shows no signs of slowing down.
What's more, once we create this data, it doesn't just sit around and remain in one place. It's moved, transmitted, sent between parties every time you stream Netflix, visit your favorite website, or just call a friend. Therein lies a problem.
You see, handling this data requires energy. As the demand for data grows, so does the energy consumption associated with it. Some worst-case models predict that by the year 2030, up to 20 percent of our global energy production could be due to the technology associated with our data.
As a PhD student in applied physics here at Harvard University, I've worked with colleagues to help realize technologies that can allow us to meet our rapidly growing demand while also reducing the energy consumption associated with it. But before I tell you about how we do that, I need to give you a little bit of a picture as to how our data is actually moved around.
Take two parties that want to communicate. They could be governments, computers, or just people. For today's example, let's use my parents, Peter and Judy. If Peter wants to send, say, a video message to Judy using his phone, well, that message can be represented digitally as a long series of ones and zeros—bits of information.
To send these bits today inside systems like your local cell tower, we actually use lasers. When the laser's on, that's a one. And when it's off, well, that's a zero.
Now, what generally limits here how quickly we can send information is how quickly we can switch between on and off, ones and zeros. In conventional systems, we use devices called modulators to perform this function. But conventional modulators have a problem. As we begin to operate them faster and faster, they need more and more energy to operate. But we need faster operation to meet our growing demand.
To tackle this problem, I focus on making modulators out of a unique material called lithium niobate. What makes this material unique is that when I send laser light through it, I can turn it from being on, passing through, to off simply by applying an electric field across the material. Now, the amount of electrical energy required to switch between on and off, ones and zeros, well, that depends on the amount of spatial overlap I can create between the light traveling through my system and the electric field I apply across it.
In conventional modulators, as you can see here, that overlap is small. As a result, the energy consumption is high. But if I could develop a way to shrink this system, such that overlap became maximized, I could drastically reduce the energy consumption of my modulator. Let me show you exactly how this is done.
That's me here at Harvard's Center for Nanoscale Systems, where I start with an ultra-thin filamentous material over 100 times thinner than a human hair. Then, using specialized instrumentation, I pattern this device with features that can be as small as a cell nucleus. Next, I etched the material using highly energetic ions traveling over 1,000 miles per hour. These ions knock atoms out of the crystal, leaving behind what I call my waveguide region, where light will be confined.
Finally, I deposit metal electrodes that allow me to precisely and locally apply electric fields where my light is. The end result is what we call a thin-film lithium niobate modulator. This device achieves the desired overlap between the light traveling through the device and the electric field I apply across it. As a result, when we compare it to conventional systems, this device consumes over a factor of 10 less energy. We are already seeing the impact of this technology in the real world.
HyperLight, a Harvard spinout commercializing this thin-film technology has shown, in collaboration with real data centers, that by using this thin-film technology, we can reduce the energy consumption of data centers by up to 20 percent. And with future engineering improvements, this number can be continued to be driven down while providing the faster data rates that we need to meet our growing demand.
So what's next? Well, the ability to quickly and efficiently control light is invaluable for technical domains outside of our data. Fields like augmented and virtual reality, biosensing, and even quantum computing are just a few of the areas where we will soon see this technology applied. And while each of these technical domains has big challenges ahead, I think that these tiny devices are up for the task.
Get the Latest Updates
Join Our Newsletter
Subscribe to Colloquy Podcast
Simplecast