Colloquy Podcast: A Cheaper Way to Make Drugs?
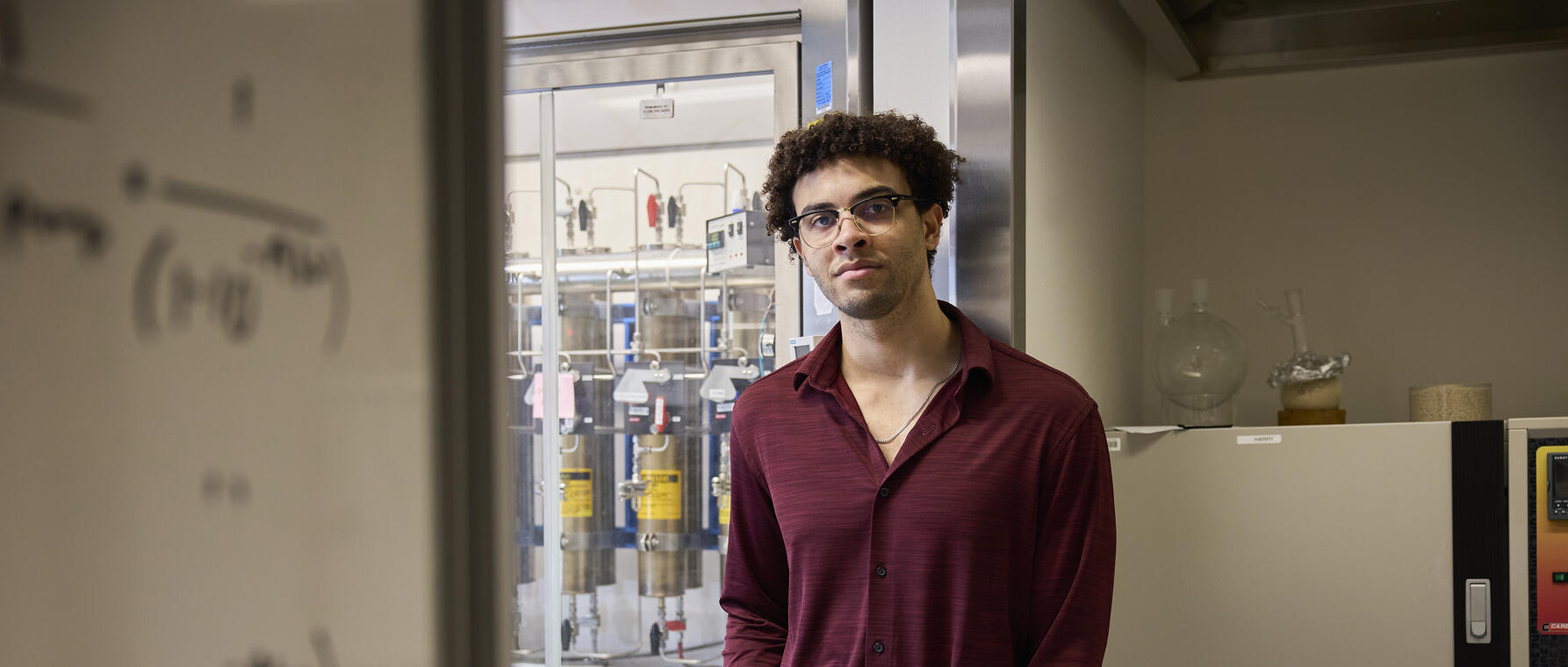
The cost of prescription drugs is high—particularly in the US where consumers pay nearly three times more than those in 33 other nations in the Organization for Economic Cooperation and Development.
One factor in prices is fluorination, which plays a crucial role in the production of many widely-used pharmaceuticals. Driven by the high cost of reagents needed for the trifluoromethyl (CF₃) group, the process is expensive—and hard on the natural environment. If there was a way to make fluorination more accessible, sustainable, and affordable—it could reshape how we approach drug synthesis—and much else in chemistry.
Chemist and Harvard Griffin GSAS PhD candidate Brandon Campbell has developed an innovative method of fluorination that could do just that. Using silver and visible light, Campbell’s pioneering approach promises a cost-effective and eco-friendly alternative to traditional synthetic methods.
Don't miss this year's Harvard Horizons Symposium in Sanders Theatre on Tuesday, April 8, 2025. Free and open to the public.
So, for people like me who might not have a PhD in chemistry, could you explain what trifluoromethyl groups are and why they're important in industry?
Yeah. The trifluoromethyl group is—it's basically a modification of the quintessential methyl group. And a methyl group is just a carbon with three hydrogens bound to it. And it's ubiquitous in natural products, from a bunch of molecules in your body, to synthetic precursors, to a whole bunch of commodity chemicals. It's very common.
The trifluoromethyl group, on the other hand, is a methyl group, which has had its three hydrogens replaced for three fluorines. And it is a very unique functional group because fluorines are kind of similar in size to hydrogen. So its steric profile hasn't changed much. And by that, I mean that it takes up a similar amount of space and a 3D conformation.
However, its properties are dramatically different than a regular methyl group because it has fluorines, which are the most electronegative atom on the periodic table instead of hydrogens. And that gives the trifluoromethyl group a bunch of properties that make it desirable for a variety of applications.
What are some of those properties that you were just talking about?
Yeah. So when a medicinal chemist is designing a drug, there's a lot that goes into it. So you have to think about: how is it going to cross the membrane to get into the cell that you want it to get into? How is it going to get cleared from the body—so, metabolic clearance? How long is it going to stay in your body—so, the half-life? All of these things have to be kind of delicately balanced to improve a drug's efficacy and also its safety. And fluorine is like a substituent that you can use to tweak these properties.
So great. Fluorination is important in the efficacy of drugs and a wide range of other properties that you can tweak. So what's the problem?
There are very, very, very few natural products that contain carbon-fluorine bonds. And there are zero natural products that contain the trifluoromethyl group. And that's because nature doesn't possess the capability to do fluorination reactions.
So basically, anytime you see a fluorine atom in a pharmaceutical, and that's over 20 percent of all FDA-approved drugs, a synthetic chemist, like myself, had to put it there because you can't get the building blocks from natural products. So this requires innovative, synthetic techniques that can be quite challenging.
So talk about that a little bit. If you can't find it in nature and you have to synthesize it, how do you do that?
To synthesize fluorinated molecules, you need a fluorination reagent. So that's some precursor that contains fluorine. And then when you run the reaction, that precursor transfers its fluorine atoms to your target substrate. And you kind of need to have these reagents be spring-loaded, for example, for reactivity.
So it should be quite easy to transfer that fluorine atom because then you don't have to put a lot of energy into the reaction to liberate those fluorine atoms. So the reaction is quite mild. And complex drug molecule precursors can be tolerated under the conditions. If you need to supply a lot of energy to liberate those fluorine atoms through a reagent, that energy can also maybe degrade the molecule that you're trying to functionalize. So that's an issue. So you want it to be quite easy.
But oftentimes, to generate these so-called spring-loaded fluorination reagents, they require a lot of steps themselves to produce. And they can be quite synthetically complex, meaning they're quite expensive themselves.
So a really common reagent is the Togni reagent. Those can be like $100,000 per kilogram, basically. So they're really, really expensive, and some of them have other drawbacks.
So one that's commonly used in industry is trifluoromethyl iodide. The drawback there is it's a gas. So it's hard to handle. And it costs a lot of energy to liquefy it and have it be in a regime in which it's easy to handle.
And then fluoroform is another gas that's really cheap. However, it's a really, really, really potent greenhouse gas. So it's actually hard to use because it's kind of banned under—I'm pretty sure it's banned under the Montreal Convention. And if not, it's just there's a lot of regulations because it's a horrible greenhouse gas.
So what my research was really focused on is a cheap alternative to these fluorination reagents, specifically trifluoroacetate or trifluoroacetic acid. It is basically acetic acid, but the methyl group has been transformed into the trifluoromethyl group. Acetic acid is vinegar. So you have it in small percentage solutions in your home, most likely.
So you're talking about household-level vinegar.
Yeah. So household vinegar is basically just a small percentage of acetic acid. And trifluoroacetic acid is acetic acid that has had its hydrogens replaced for fluorines to make a trifluoromethyl group. It's quite easy to make, and it's made on a multi-ton scale annually. And for that reason, it's really cheap. However, it's hardly used for fluorination reactions.
So why?
The main problem to using trifluoroacetate is just that I have to oxidize it in order to use it, and that's really, really hard. You have to use temperatures of 160 degrees Celsius or higher, or you have to apply voltages in excess of two volts. And those conditions are likely to destroy the molecule that you're trying to functionalize with the trifluoromethyl group. So it's hardly used just because the reaction conditions you have to use—employ—are too harsh.
So you destroy what you're trying to make.
Yeah. If I have to apply two volts to oxidize trifluoroacetate, most molecules can't themselves withstand two volts, and they will also get oxidized.
You use something called photoredox with silver photocatalysts. Can you describe the process? And what makes it a breakthrough in activating trifluoroacetic acid?
Redox chemistry is just any sort of chemistry in which there is a transfer of charge. If something gains an electron, that's called reduction. If something loses an electron, that's called oxidation. So redox is just reduction-oxidation together—redox.
And photoredox is the triggering of redox reactions using light, using photo illumination. Light is an amazing energy input because it's quite mild. So the light that's lighting up the room that we're sitting in right now can be used to drive chemical reactions.
I have something that can absorb light, a catalyst, like a photocatalyst. And once it absorbs light, it becomes much better at either ripping electrons off of things or giving electrons to things. So I basically use light energy to drive the redox chemistry.
So naturally, you would think, can I use photoredox to oxidize trifluoroacetate, to use it for fluorination reactions? But most, if not all, of the traditional photocatalysts available, they didn't have enough oxidation power to do that transformation. For that, I would need to bind TFA to some metal complex. And then when you shine light on that, the electron will jump from the TFA to the metal center.
I knew I would need a high-valent, kind of unstable metal that really wants to decrease its oxidation state to achieve a more stable configuration, and in that context, would be able to oxidize TFA. And that's why I turned to silver. Silver zero is silver metal. I'm wearing silver jewelry right now. Silver is very common—silver coins.
Silver 1 plus is a very common silver oxidation state. Silver nitrate, silver 1 compounds—doctors actually used that to cauterize your nose. Silver 1 bromide is another very common silver 1 salt. That was used in the first cameras, believe it or not.
But there's a simple question. What if I take a second electron from silver to make silver 2 plus? That is incredibly unstable. So it's really hard to make these silver 2 compounds. And even worse is when you do make them, they actually tend to be like rocks, basically. And by that I mean they don't dissolve in many common solvents that we use to do solution state chemistry.
And just from looking at that, I figured it would be the perfect metal center for liquid metal charge transfer because it wants that electron so bad, that I imagine that if I could bind basically anything to a silver 2 center and shine light at it, I should be able to get the charge transfer to happen.
But obviously, the main hurdle that needs to be cleared here is, how do I make these silver 2 compounds? And how do I tame them enough so that they're actually easy to handle and easy to use? And once I had this compound, it was—I just needed to test a hypothesis.
So if you dissolve it into an organic solvent, such as acetonitrile, and you add trifluoroacetic acid, then you make a new compound. And what I actually found was I made two different molecules.
So the first one was where one trifluoroacetate binds. And that's like this yellowish compound. And then when two bind, it turns orange. So now I had these compounds in which trifluoroacetate was bound, and in the dark they're stable so I can isolate them.
But then when you expose them to light—so simple LED illumination—you quickly see the color disappear, and they turn colorless, which is an indication that I formed silver 1. And if I use various chemical techniques, such as nuclear magnetic resonance spectroscopy, I saw evidence of trifluoromethyl radical reactivity, meaning that I oxidized the TFA, decarboxylated it, generated the trifluoromethyl radical, and then that just went and reacted with stuff. So the next step to that was adding in a substrate to trifluoride methylate.
That you want to fluorinate.
Yeah. So the simple one is like, I just toss in benzene, which is just six carbons in a ring. And then I add in my silver 2. I add in trifluoroacetate. And then I expose the solution to blue light. And then afterwards, I recover trifluorotoluene, which is the product that you would expect if you trifluoromethylate benzene.
So that was a really cool find. It was really cool that you were able just to take these salts that you have and then shine light on them with trifluoroacetate and get this trifluoromethylation reactivity.
What are some other potential applications of your method?
What I think is really striking about this method is it doesn't require high-energy light to get the transformation to proceed. While it's really fast with UV light, it's still fast with purple light. It's still fast with blue, and it's still fast with green. And it can even happen with orange and red light, although it gets quite slow at that point.
So it's been a huge area of active research in photochemistry—is using lower energy light to do these transformations because it's less of an energy input. And also, lower energy light penetrates into reaction media better than higher energy light. So for industrial applications, you actually want lower energy light so that you can evenly irradiate your reaction mixture.
And then I'll say another cool thing is because of how powerful silver 2 is as a so-called ligand-metal charge transfer chromophore, we were able to oxidize one of the hardest things to oxidize, which is trifluoroacetate. So then that begs the question, What else can you oxidize? If I can oxidize trifluoroacetate, I should be able to oxidize a lot of other things.
So currently, in our group, it's a very active research area where we're targeting other substrates that are of relevance to a variety of sectors, such as pharmaceuticals or just commodity chemicals, and undertaking these oxidations using silver 2 catalysis and light.
So I'd say that was what kind of intrigued me about this project the most, is just using silver in a context that's never been used in before. Silver 2 is very rare in the chemical literature, and it has never been used as a photo reagent. So we kind of like crossed that boundary and showed that it was possible.
And while it's still sort of like a novel, kind of far from an applicable type of platform, I think it has demonstrated there's a lot of real power there to be exploited with further work in the area.
So finally, kind of “big picture” it for us. Why does the work matter? And why is it so important to make CF3 incorporation more cost effective and sustainable, not just for the pharmaceutical industry, but for other applications as well?
Why does it matter is over 20 percent of drugs contain fluorine. Not all of those are in the form of the trifluoromethyl group. The trifluoromethyl group is just a decently sized piece of the pie.
But ultimately, I'm not a businessperson, so I don't know how drugs are priced. I'm just a scientist. But I would imagine that the price of a drug will increase based off of how expensive it is to itself make. So if you have to rely on really expensive fluorination reagents to make the drug, that price is probably going to translate to consumer, I would imagine.
So it's always a goal in chemical synthesis to take really cheap and abundant and sustainable precursors, those that are made on a really large scale with a small carbon footprint, and to be able to use those as chemical feedstocks for your reactions is very important.
So I would argue that trifluoroacetic acid is probably the ideal trifluoromethyl source. And it would obviously be a great thing if it was used in that context more often.
This research was funded by the National Science Foundation, the National Institutes of Health, and the U.S. Department of Energy.
Don't miss Brandon at this year's Harvard Horizons Symposium in Sanders Theatre on Tuesday, April 8, 2025. Free and open to the public.
Banner photo by David Salafia
Get the Latest Updates
Join Our Newsletter
Subscribe to Colloquy Podcast
Simplecast