The Alchemist
Transforming elements into superconductors, atom by atom

The Greek mathematician Archimedes probably never ran naked into the streets of Syracuse crying “Eureka!” (“I’ve found it!”) after discovering the law of buoyancy and volumetric displacement while lying in a bathtub. Nevertheless, most scientists work in the hope that they will have their Eureka moment—a day when they make a discovery that changes the way scientists in their field approach an important problem. For Grace Pan, that moment came in the fall of 2020 while she was working at Harvard’s Laboratory for Integrated Science and Engineering (LISE).
“I measured this compound I synthesized,” she remembers. “I didn’t think it would work. I let the data sit in my computer for a whole day before I even looked at them. But then I plotted them out and checked them. And then I’m suddenly yelling ‘More helium! I need more liquid helium to confirm this data!’ I couldn’t believe it was happening.”
What was happening was that the PhD student in physics at Harvard’s Graduate School of Arts and Sciences (GSAS) had created a new superconductor, a material that conducts electricity with no resistance when cooled below a certain temperature. (Hence the calls for liquid helium.) Moreover, she had done it through a kind of modern-day alchemy, building the material atom by atom from the elements neodymium, nickel, and oxygen in a way that gave the resulting composite new properties.
The young physicist isn’t stopping there. Building on her 2020 discovery, Grace Pan hopes to construct a new generation of high-temperature superconductors (HTS) that could deliver cheap, abundant power and perhaps even help solve the climate crisis.
Beyond Nature
In a traditional conductor—copper wire, for instance—energy lost to electrical resistance is released as heat. It’s why the servers in huge data centers need to be kept constantly in a cool environment. The electricity needed to run them generates so much heat from resistance that it could damage the equipment and the data that it stores.

Moreover, that loss of energy adds up. An estimated 100 billion to 200 billion kilowatts of energy are dissipated by servers every year—about two or three percent of the annual energy consumption in the United States. In countries with less efficient electrical grids, the loss of power during transmission is even greater.
With a superconductor, electrical resistance drops to zero. The quantum mechanical properties of the material enable electrons to move without colliding with the ions that vibrate in a traditional conductor like copper wire. That loss of resistance shrinks energy loss to nothing, a massive increase in the efficiency of transmission.
Unfortunately, for superconductors to function in this way, they must be cold. Really, really cold. As cold as that liquid helium Pan was shouting for in September 2020: -452.11 degrees Fahrenheit. Cooling substances like liquid helium are expensive and difficult to produce, making most superconductors impractical for common use.
Pan is part of a generation of physicists working to create a superconductor that functions at higher temperatures. To do this, she works with layered oxides: oxygen-based materials similar to minerals but made synthetically through a process called molecular beam epitaxy. Pan places an element like titanium or calcium in an ultra-high vacuum chamber and heats it as high as 1,200 degrees Celsius. At high temperatures, the atoms begin to evaporate off the element much like steam off of a pot of boiling water. As they do so, a series of shutters open and close to allow or prevent atoms from landing on a surface at the top of the chamber. This enables Pan to build a material atomic layer by atomic layer.
“We're evaporating these atoms, turning them on and off very, very precisely,” she says. “We end up with a single layer of calcium atoms, a single layer of titanium atoms, a single layer of calcium atoms, etc. It allows us to bypass a lot of the rules that govern chemical reactions by placing atoms exactly where we want them to be.”
To oxidize the elements, Pan conducts this process in a highly reactive, distilled ozone environment that is exponentially more reactive than simple molecular oxygen. This enables her to essentially force oxygen into the elements she uses to stabilize their atomic structure.
“Imagine if you held a pan over a pot of boiling water,” she explains. “You know the steam is going to rise up and hit the pan. As the atoms from those super-hot elements evaporate, we have an ozone nozzle pointing at the surface they land on. When the atoms hit that substrate, they encounter the ozone atoms and oxidize right there.”
The advantage of this process, Pan says, is that it not only allows scientists greater control over the materials they create but also enables them to combine elements in ways that would never occur in nature.
“We can be really creative,” she says. “For example, we can have single layers of calcium and titanium, then put a double layer of, say, calcium. Or I could build with 10 layers of lanthanum manganite and 10 layers of calcium titanate. I can make ‘sandwiches’ of different materials that are atomically precise.”
Laying It On
The layering method not only creates new materials but also creates materials that have new properties. For instance, neither lanthanum aluminate (lanthanum, aluminum, and oxygen) nor strontium titanate (strontium, titanium, oxygen) is a good conductor of electricity. If you layer them together, though, the places where the materials meet exhibit superconductivity.
“These properties just come out of the woodwork,” Pan says. “That layered interface is where they emerge. It’s an amazing feature of this layering technique—to engineer and create where there was nothing before.”
The property Pan wants to elicit from the materials she makes is superconductivity at temperatures that don’t require expensive and difficult cooling. But rather than research the most popular superconductors—copper-based oxides called "cuprates"—Pan decided to focus on nickel instead. Right next to copper on the periodic table of elements, nickel has a chemical structure very similar to that of its superconducting neighbor. Nonetheless, nickel never exhibited superconducting properties until recently, when researchers at Stanford combined the element with neodymium, oxygen, and a small amount of strontium. Even so, the nickelate material needed to be chilled to temperatures much lower than cuprates to superconduct.
Pan says that the method the Stanford team used in their nickelate was akin to making casserole: they blended many ingredients together to get something new. Her approach resembles the process for a different kind of baked dish.
It’s an amazing feature of this [atomic] layering technique—to engineer and create where there was nothing before.
“We basically make a lasagna with a double lasagna noodle of neodymium every five layers,” she says. “We start with a layer of neodymium and a layer of nickel. We do this four more times for a total of five layers of neodymium alternating with five layers of nickel. However, after each block of this ‘five-layer’ compound, we insert two layers of neodymium. So we have a double layer of neodymium sandwiching every five layers of neodymium nickelate. Both elements oxidize when they evaporate up, land on the substrate, and encounter the distilled ozone. This method enables us to tune the material to superconduct simply by working with the dimensionality of the system.”
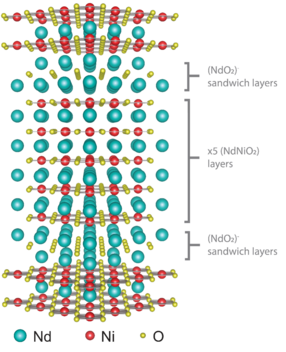
The nickelate material Pan created, described by the chemical formula Nd6Ni5O12 or schematically as (NdNiO2)5(NdO2), marked the first time that anyone had ever elicited superconductivity through this type of structural engineering alone. And although her nickelate “lasagna” did not superconduct at a higher temperature than a cuprate, Pan feels optimistic about the possibilities of the technique.
“The creation of the material was only one part of our work,” she says. “The other part was predictive. Our research suggests that if we shrink the number of layers from five to two or three, and if we can synthesize it at high quality, the compound should superconduct even more—and at a higher temperature.”
HTS could make possible electrical grids that lose no energy in transmission, high-speed supercomputers, even superfast trains that travel on a bed of magnetism rather than metal tracks. Their greatest promise, however, may be their potential for use in nuclear fusion reactors that could generate nearly limitless energy with no direct greenhouse gas emissions, no long-lived radioactive waste, and no danger of meltdown. Fusion reactors need powerful magnets to confine the plasma in which the nuclear reactions take place. Powerful magnets need the kind of electricity that only material with no resistance can deliver. The development of a superconductor that would not require extreme cooling could make fusion power a reality.
“With a superconductor, you can send an enormous amount of current through a wire," Pan explains. “That enables you to generate a large magnetic field that takes little power to maintain. If we can make an HTS that doesn't require so much cooling power, it would not only be practical to build and operate fusion reactors but also to replace all the conductors we now use. It would be remarkable.”
If we can make a high-temperature superconductor that doesn't require so much cooling power, it would not only be practical to build and operate fusion reactors but also to replace all the conductors we now use.
Now in her fourth year at GSAS, Pan hopes to continue her research after graduation and work with students like her undergraduate mentee Denisse Córdova Carrizales, who recently produced a video on how superconductors could solve the climate crisis. Wherever she lands, Pan will continue layering atoms in her search for a high-temperature superconductor and other new materials.
“I’d be thrilled to be faculty at a university,” she says. “But honestly, I want to do whatever enables me to continue mentoring, teaching, and doing this science. I hope that in my lifetime we will find an HTS, make fusion power practical, and begin to mitigate climate change. I want to be a part of that.”
Photos by Tony Rinaldo
Get the Latest Updates
Join Our Newsletter
Subscribe to Colloquy Podcast
Simplecast Stitcher