Hydro Heidi
Heidi Pickard tracks down the toxic ‘forever chemicals’ that contaminate surface waterways
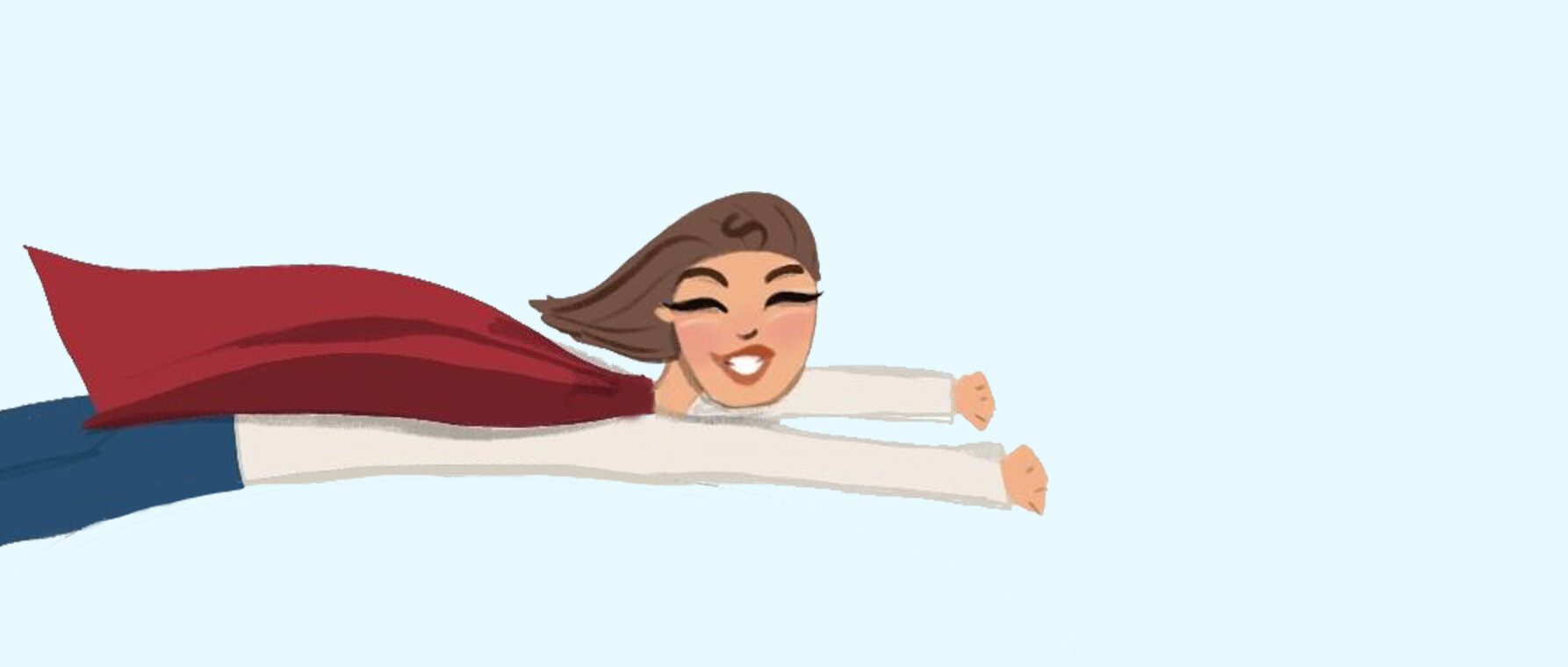
You should know three things about per- and polyfluoroalkyl substances (PFAS). The first is that these chemical compounds are persistent. The strength of their bonds prevents them from breaking down in the natural environment. The second is that they’re everywhere; PFAS are used in carpeting, furniture, cosmetics, and many other consumer products. The last and probably most important thing to know is that PFAS are probably bad for you. The chemicals are associated with widespread negative impacts on health.
“[The scientific community has reached] good consensus that PFAS exposures are associated with certain health effects,” says Heidi Pickard, an engineering sciences PhD student at Harvard’s Graduate School of Arts and Sciences (GSAS). “Some of these effects include liver disease, decreased fertility and hypertension in pregnant women, immune and developmental effects in children including decreased antibody response to vaccines, and certain organ cancers.”
The good news is that private industry is shifting away from the production of certain types of PFAS—largely in response to regulation like the PFAS Strategic Roadmap recently announced by the Environmental Protection Agency. The bad news is that the shift is usually to different kinds of PFAS. Alarmingly little is known about these substances, referred to as PFAS precursors. That’s why Pickard—nicknamed “Hydro Heidi” by a friend who chronicles her efforts in cartoon form—seeks to uncover through her doctoral research the prevalence of these PFAS precursors in surface waterways, as well as their impact on the environment and health.
The Problem with PFAS
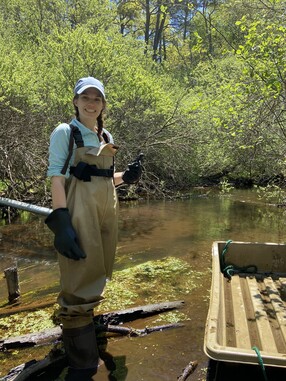
Per- and polyfluoroalkyl substances are a class of organofluorines (OF), organic compounds that contain a carbon-fluorine (C-F) bond. This bond gives PFAS some unique properties. The fact that they repel oil and water, are stain-resistant, and non-stick, for instance, make PFAS attractive for use in consumer products that range from carpeting and furniture to food packaging, outdoor gear, cosmetics, cookware, and aqueous firefighting foam. But that C-F bond is also what makes PFAS so difficult to get rid of.
“The C-F bond is one of the strongest bonds in nature,” she says. “That makes these compounds very resistant to breaking down in the natural environment. They’re very persistent under normal environmental conditions. That’s why they’re called ‘forever chemicals.’”
Because PFAS don’t break down, they build up in the environment and eventually in our bodies through the food we eat, the water we drink, the air we breathe, and the things we touch. They can even be transferred from mother to child through breastfeeding. Pickard says that the levels at which PFAS accumulate in humans and animals can be associated with troubling health effects as indicated in many laboratory studies. “The Agency for Toxic Substances and Disease Registry just published in 2021 an extensive review of epidemiological studies for 14 PFAS compounds,” she notes, “summarizing the associated negative health effects with them.”
The carbon-fluorine bond is one of the strongest bonds in nature. That makes these compounds very resistant to breaking down in the natural environment....[It's] why they’re called ‘forever chemicals.’
—Heidi Pickard
Moreover, because PFAS are so persistent and so widely used, the contamination problem is widespread. Pickard says that PFAS have been detected not just at industrial sites and adjacent areas, but also in the high Arctic, the Antarctic, and remote islands—all locations far from sources of contamination. The PFAS Project Lab at Northeastern University created a map that identified almost 58,000 sites in the US suspected of PFAS contamination. An estimated 98 percent of the US population has detectable levels of the chemicals in their blood.
“PFAS are released into the air from manufacturing and can transport long distances,” Pickard says. “They leach into the soil and groundwater from consumer products or industrial waste in landfills and from fire-fighting foam. The effluent and biosolids from wastewater treatment processes that don’t remove these chemicals get released into waterways and contaminate farmland. These are just some of the ways that these chemicals get into our groundwater, drinking water, surface waters, soil, and air. They then get taken up into plants and accumulate in biota. Anywhere you look for PFAS, you’ll probably find them.”
Searching for Precursors
Not surprisingly, PFAS have increasingly become a concern for environmental regulators in recent decades. The PFAS Strategic Roadmap announced by the Environmental Protection Agency in October 2021, for example, commits to new research, regulation, and remediation of PFAS contamination. In response, industries have shifted to using polyfluorinated substances that are usually PFAS precursors. Unlike terminal PFAS, precursors do break down, which allows manufacturers and others to evade regulation. But the degradation is only partial, leaving the precursor’s terminal PFAS component in the natural environment.

“The problem is that we don’t know what these PFAS precursors are,” Pickard says. “We don’t have analytical standards to quantitatively determine if they are in the environment and at what levels. They’re harder to analyze or detect with our current methods. We have little to no information on the toxicological effects of these compounds.”
That’s where Pickard’s research comes in. She’s currently looking at the accumulation of PFAS in fish and other seafood from surface waters both in southern New Hampshire and Cape Cod, Massachusetts, to better understand the extent of contamination and exposure from PFAS precursors.
“Some precursors are persistent enough that they stay in the water or sediment and accumulate in fish,” she explains. “Most will eventually biotransform to the terminal PFAS but we don’t know on what timescales, under what conditions, and if it preferentially occurs in certain organs of different species. We also don’t know much about the health effects of most precursors, but we do know about the effects associated with terminal PFAS. So, if these precursors accumulate and biotransform to the terminal PFAS, then we know there’s an increased probability for exposure and possible toxicity.”
The challenge for researchers like Pickard has been that targeted mass spectrometry methods, the most common technique for measuring PFAS accumulation, capture only a small fraction of the substances used in commerce and released into the environment. “Thousands of PFAS exist, but we only have analytical standards to target about 40 of them, few of which are PFAS precursors,” Pickard says.
The problem is that we don’t know what these PFAS precursors are...We have little to no information on the toxicological effects of these compounds.
— Heidi Pickard
Instead of using only standard mass spectrometry, Pickard has innovated a multi-method approach to her search for precursors. She takes a sample—say, a piece of fish—and extracts the PFAS using different solvents and extraction techniques. Then she analyzes the extracted sample using different instruments, each of which provides bits of information about the concentrations and chemical structures. Combustion ion chromatography (CIC) enables Pickard to determine the amount of organofluorine in a sample. That information can, in turn, reveal how much organofluorine is present.
“Organofluorine in environmental samples consists of both PFAS and other organofluorine chemicals such as certain pesticides and pharmaceuticals,” Pickard says. “CIC tells us about the total amount of PFAS that could be in the sample.”
However, CIC doesn’t tell Pickard which PFAS compounds are present. For that information, she uses several other methods. Targeted mass spectrometry captures the fraction of the sample that consists of known PFAS. The remainder probably consists of PFAS precursors. Using both non-targeted mass spectrometry and other extraction and statistical methods, Pickard identifies which precursors are present and quantifies their concentrations in the sample.
“You need a toolbox of many different methods to get the whole picture of PFAS,” she says. “Just one method won’t tell you everything you need to know with these chemicals now.”
Using this process, Pickard analyzed the muscle tissue of eight species of recreational freshwater fish caught in southern New Hampshire. She found traces of PFAS in all samples originating from precursors associated with the electrochemical fluorination (ECF) process historically used in manufacturing. She says the substances have “a potentially high bioaccumulative potential,” meaning that they will keep accumulating in the fish over time.
“The results generally indicated that ECF-based precursors, particularly a class of short-chain perfluoroalkyl sulfonamides, are present in these fish samples, meaning that this class of precursors is both persistent and bioaccumulative,” Pickard says. “Future analyses should target them to accurately quantify their contribution and assess the full extent of exposure to recreational fish consumers. People rely on these fish for food. We don’t know what levels of exposure to these precursors could be a concern but identifying their presence and bioaccumulative potential is the first step.”
In addition to understanding how precursors behave and accumulate in biota, my results will hopefully also be used by local or state governments to establish necessary fish and water advisories in their areas for risk mitigation.
— Heidi Pickard
Pickard's advisor Elsie M. Sunderland, professor of environmental science and engineering, says that exposure to PFAS is often underestimated because of a failure to take precursors into account. Her advisee’s research will fill that gap.
“Heidi is investigating less understood exposure pathways to PFAS and also the potential for precursors to bioaccumulate in food webs,” she says. “Her work has direct relevance to regulations for these compounds such as fish consumption advisories and water quality protection. It’s also pushing communities to think about new ways to detect and report these compounds when they are present in the environment.”
Pickard’s research will also provide a toolbox of analytical methods that will enable researchers to better assess the full extent of PFAS contamination in the environment and to link the transport of PFAS from source sites to fish consumed by wildlife and humans—particularly members of indigenous communities who rely on their local catch as a food source. She hopes that understanding how these toxic chemicals accumulate and behave in the environment will enable local officials to better mitigate the risk that PFAS pose.
“In addition to understanding how precursors behave and accumulate in biota,” she says, “my results will hopefully also be used by local or state governments to establish necessary fish and water advisories in their areas for risk mitigation. My hope is also that more agencies will start thinking about precursors as an important part of our PFAS exposures. We must account for precursors when assessing biological exposure to PFAS, especially if a lot of these precursors are entering food chains, bioaccumulating in organisms, and biotransforming to substances that have known toxicological effects.”
Photos courtesy of Heidi Pickard; Banner Illustration by Meredith Wish
Get the Latest Updates
Join Our Newsletter
Subscribe to Colloquy Podcast
Simplecast